India currently stands in a moment of opportunity in which it is evident that transitioning to low-carbon systems can bring about economic growth. The falling costs of renewable energy, ambitious government plans for rapid deployment of renewable energy, and increasing financial support from international governments and investors all indicate that the transition to a low-carbon economy is not only possible, but already underway.
As a part of the 2015 international Paris Agreement on climate change, India has pledged a reduction in emissions intensity of 33-35% by 2030 from 2005 levels. India has also set a target to transition to non-fossil fuel based energy for 40% of its cumulative electricity generation capacity by 2030. However, the work remains to figure out the practicalities of the low-carbon transition at the national, sectoral, and industrial levels. One key sector of the Indian economy that could set a strategic example for decarbonization and meeting India’s 2030 targets is the rail transport system, and specifically Indian Railways (IR), India’s national railway service.
IR is currently the world’s second largest railway network and is the single largest consumer of electricity in India, consuming about 18 TWh per year, or roughly 2% of the country’s total power generation. IR also consumes 2.6 billion liters of diesel annually, or 3.2% of the total diesel consumption of the transport sector in India. In addition, the energy demand of IR is expected to triple by 2030 to 49 TWh due to increasing passenger volume.
Because of the volume of IR’s energy consumption, prioritizing decarbonization of Indian Railways could help India achieve its 2030 emissions reduction goals as well as improve energy security by reducing fossil fuel imports. In addition, decarbonization may be a more cost-effective option that is cheaper than the business-as-usual scenario for IR in the long run.
In this report, we have identified different potential pathways to decarbonization of IR by 2030 and examined their cost-effectiveness and feasibility.
A first and essential step to decarbonization of IR is to transition to an electrified rail network, and away from a diesel-powered rail network. As of 2015, IR used predominantly coal-based power and diesel fuel. It had electrified 38% of its track (in route kms), which carries approximately 63% of freight traffic and 50% of passenger traffic. An electrified rail network can more easily transition to clean energy alternatives such as solar and wind power, whereas there is limited availability of clean fuel alternatives. In line with this, IR already has aggressive plans for electrification.
Assuming electrification to the maximum extent possible, we have identified and examined eight possible decarbonization pathways separately for the traction segment (energy use for the railroads) and the non-traction segment (energy use for the supporting infrastructure: the stations, service buildings, street lighting, etc.) of IR. The different scenarios we considered are: captive generation (where IR builds and owns renewable energy capacity) vs. purchasing renewable energy; at a normal rate (100% decarbonization by 2030) vs. at an accelerated rate (100% decarbonization by 2020); and an all solar power pathway vs. a mix of solar and wind power.
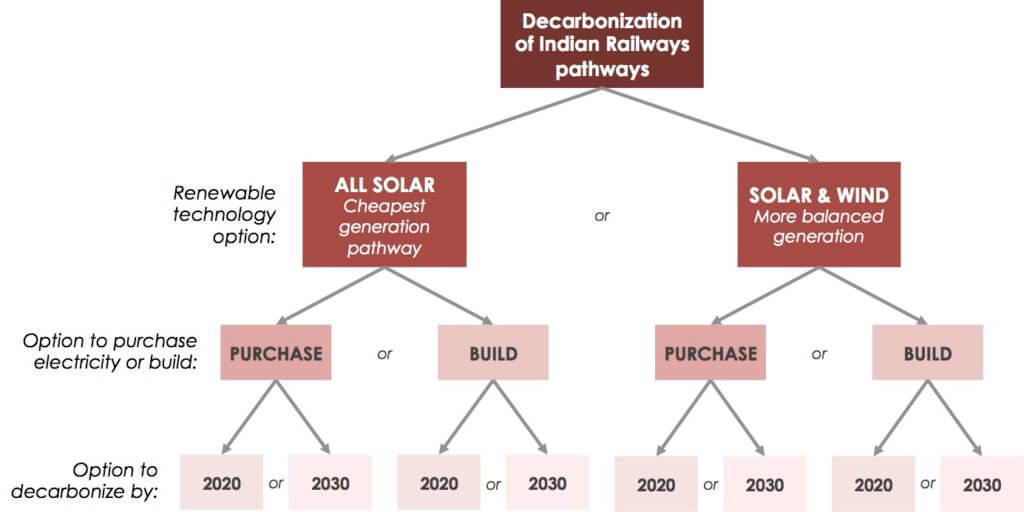
In order for decarbonization of IR to be successful, it’s necessary for the pathway to decarbonization to be both cost-effective and feasible to implement. We have examined the cost-effectiveness of each pathway, as well as potential barriers to implementation.
Solar and wind power are the most feasible clean electricity options for IR, because other renewable sources have much longer construction times. While an all-solar pathway would be cheaper in terms of pure generation costs, a mix of solar and wind would provide a more balanced generation profile, and thus lower balancing costs. As we found that an all solar pathway is only 6% cheaper than a mixed solar and wind pathway, and a mix of solar and wind power might have lower overall costs due to lower balancing costs, we calculated the costs of each decarbonization pathway assuming a mix of solar and wind power.
To assess cost-effectiveness, we compared the costs of each decarbonization pathway (which includes all costs, except balancing costs) with a business-as-usual scenario, where IR does not decarbonize at all, in both net present value terms and yearly cash outflows. Because the costs of power are different for the traction and non-traction segments under the business-as-usual scenario, we analyzed the two segments separately.
For the traction segment, we found that all decarbonization pathways are more cost-effective than business-as-usual, and that the most cost-effective pathway is captive generation, at an accelerated rate of decarbonization. When we examined yearly cash outflows, we found that the accelerated, captive generation pathway is not only the cheapest on average but also the least volatile; it is 32% cheaper and 66% less volatile than business-as-usual. Furthermore, we found all decarbonization pathways to be at least 17% cheaper than business-as-usual. Even in net present value terms, we found all four options to at least 15% cheaper than business-as-usual, which establishes a clear case for 100% decarbonization by 2030 in order to lower costs.
For the non-traction segment, we found that the decarbonization pathway of captive generation at an accelerated rate of decarbonization is even more cost-effective than in the traction segment. When we examined yearly cash outflows, we found that the accelerated, captive generation pathway is 69% cheaper than business-as-usual. Furthermore, in net present value terms, all decarbonization pathways are at least 33% cheaper than business-as-usual, with the accelerated captive generation pathway providing a 50% cost savings over business-as-usual. Given the stronger greater potential for cost savings in the non-traction segment, we recommend that IR prioritize the decarbonization of the non-traction segment.
Decarbonization of IR by 2030 will lower costs by at least 17% in the traction segment, and at least 33% in the non-traction segment, when compared with business as usual.
While it’s evident that decarbonization of IR is more cost-effective than a business-as-usual pathway, it’s also important to examine if decarbonization is feasible to implement. There are several challenges to implementation of decarbonization that will be important to address, particularly poor implementation of state policies around net metering and open access, and the need for low-cost and feasible balancing options for renewable energy.
State policies around net metering and open access, which facilitate load balancing and third-party power procurement, vary state by state and are often poorly implemented. This could become a significant barrier to implementation of IR’s decarbonization pathway, specifically in the non-traction segment.
To manage issues around net metering policies, IR should enter into net metering arrangements with states which have already encouraged net metering, particularly Tamil Nadu, Delhi, West Bengal, and Andhra Pradesh. Similarly, to manage issues around open access policies, IR should aim to procure power from independent power producers in the states that have already successfully implemented the open access policy.
Further, because solar and wind power can be intermittent and variable, they will require load balancing, which requires use of technologies such as energy storage to ensure consistent supply of electricity that can meet the demand. However, not all of the technologies available for load balancing are currently feasible for IR. We examined the different load balancing options that are or will be) available to IR and assessed their technical, regulatory, and commercial feasibility. The most feasible options of the ones that are immediately available are power banking and net metering with state DISCOMS (in certain states). Pumped hydro storage may also be feasible. In the next five years, additional feasible options for load balancing will be flexible thermal power plants, grid-scale battery storage, and trading on power exchanges.